History of Perovskite
Perovskite was first found in 1839 in chlorite-rich skarn in the Ural Mountains. The part called CaTiO3 was named after Russian mineralogist Lev A. Perovski. Perovskite is a solid structure that all materials with this structure share, even if they are made of different things and have different features. Many metal oxides with perovskite structures, like BaTiO3, PbTiO3, and SrTiO3, have been studied over the years. A lot of them have ferroelectric or piezoelectric qualities. Wells made a group of lead halide chemicals with the general formula CsPbX3 more than 50 years after oxide perovskite was first found. Later, it was shown that these metal halides have a perovskite structure called ABX3. This structure is cubic at high temperatures and changes to a tetragonally twisted structure at lower temperatures. People are interested in studying electrical properties and the idea of adding organic molecules because of CsPbX3’s photoconductivity that can be changed. Mitzi et al. made a lot of organic-inorganic halide perovskites at the end of the 20th century. These materials have a wide range of shapes and physical qualities in optoelectronic, solar, ferro- and antiferromagnetic, and nonlinear optical fields.
Structure of Perovskite
One type of perovskite is ABX3, which is a crystalline family. A and B are cations, and X is an anion. In a perfect perovskite, the structure is a cube, with B cations forming a six-fold coordination ring around an octahedron of X anions and A cations forming a twelvefold cub-octahedral coordination. In 3D perovskites, the six anions at the ends of the octahedra share space with the six octahedra that are closest to them. Pb is in the middle. Large cations change the structure so that not all six halides can be shared with other octahedra. This creates 2D, 1D, or 0D materials that are inspired by perovskite.
For the perovskite structure to form, a number of conditions must be met. For example, the valencies of the A and B cations must add up to three times those of the X anion in order to keep the charge balance. Because of size limits between ions, the perovskite structure can only handle certain combos of ions. This is to protect the anion-corner-sharing structure. The Goldschmidt tolerance factor, which is linked to the ionic radii rA, rB, and rX, shows this relationship between ionic size and shape.
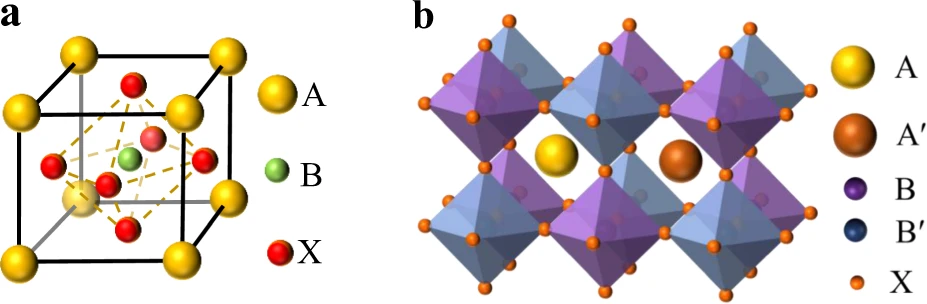
These days, many different types of perovskite structures have been made. They are all based on the original 3D perovskite structure, which is based on the corner-sharing BX6 structure. The ABX3 perovskite structure is very limited, but the low-dimensional perovskite structure and makeup can be changed in more ways. The size limits for the A′, which is the intermediate cation, are lifted for low-dimensional derivatives when the 3D perovskite is thought of as being cut into slices.
It is made up of a cation monolayer or bilayers that switch places with sheets of the corner-sharing BX6 octahedra in 2D perovskite. The 2D perovskite has either one or two diammonium cations A′. These are sometimes called Dion–Jacobson (divalent A′) or Ruddlesden–Popper (monovalent A′) phases. Other low-dimensional perovskite derivatives have BX6 links that are much more spaced out. These include 1D BX6 octahedra connected chains that look like “pillars” and 0D octahedra that are detached and look like “dots.” The BX6 connection can be split up even further by the various mixtures that make up the mixed perovskites known as fake members.
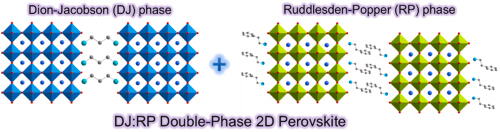
Properties and Applications of Perovskite
Halide perovskites are interesting semiconductors because they have many useful features, such as a bendable structure, high absorption, long carrier lives, and the ability to fight flaws. Because of these benefits, halide perovskite works well in lasers, solar cells, LEDs, photodetectors, and memristors. It’s interesting that halide perovskite devices can also be made on flexible surfaces in the form of polycrystalline thin films at low processing temperatures.
A lot of people are interested in organic-inorganic halide perovskites because they work very well and don’t cost much in next-generation PVs. This means that these materials can now compete with commercial thin-film solar cells. Since these materials are very good at absorbing both visible and near-infrared light, they can use solar irradiation to make energy. In 2009, the first report of a perovskite solar cell was published. Now, the highest power conversion efficiency (PCE%) of a perovskite film on a lab scale has been confirmed above 26%.
Perovskite film-based solar cells are easier to make at low temperatures, use less energy, and are better for the environment than silicon wafer-based solar cells. They also have a shorter payback time but less long-term stability. But the process of making perovskite-based solar cells more widely available has been slowed down by problems with their stability. These problems include damage from water, air, heat, light, mechanical stress, and reverse bias. These flaws don’t take away from how great the work is overall; instead, they make researchers want to find ways to make devices more stable. Halide perovskite materials are still a cheap way to deal with large amounts of electricity.
It is very clear what colour halide perovskites used as LEDs are, with a full width at a half-maximum of 20 nm for the blue or green–blue electroluminescence spectrum peaks. In contrast to other artificial nanomaterials, quantum-well nanoparticles keep their very pure colour no matter what size they are. So, halide perovskites might be able to fix some of the problems with current LEDs, like how hard they are to make, how expensive they are, how poorly organic LEDs preserve colour, and how high the ionisation energy is for quantum dot LEDs. Since perovskite LEDs were first shown in 2014, their external quantum efficiency (EQE) has quickly grown from less than 1% to 25.8% for red [19], 28.1% for green [20], and 14.8% for blue [21].
Halide perovskite-based photodetectors work just as well as widely available photodetectors made of solid Si and III–V, which means they have a lot of promise for use in light signal recognition technology. Halide perovskites have great optical properties, like photoinduced polarisation, high drift mobilities, and effective charge collection. These properties have helped the development of cutting-edge material studies in the field of light signal recognition. This group of semiconductors could help make photodetector technology that is completely new in images, optical communications, and biological sensors.
Besides the uses listed above, halide perovskites can also be used in a lot more areas because of their unique properties. These include lasers, X-ray detectors, waveguides, scintillators, gas sensors, spintronics, and photocatalysis.
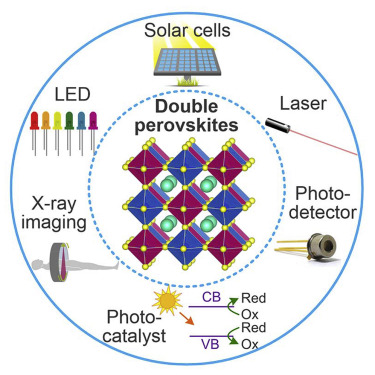
Summary and Outlook
In the last 20 years, halide perovskite materials have grown quickly. Scientists have been studying how their structure and properties are related by looking at their different phases and compositions. These materials have been used for many things, like photovoltaics, screens, sensors, and storage. But problems with environmental and thermal stability stop their progress. In order to solve these problems, scientists are looking into changes in structures and the links between parts and qualities using multimodal characterization, in situ technology, and AI technology. The halide perovskite is a treasure chest, and working together with people from different fields should lead to even more surprising findings.
Leave a Reply